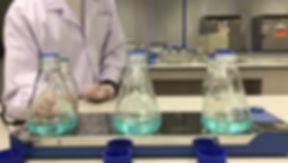

Why quantum dots have PL properties?
Abstract
ZnS nanoparticles co-doped with Cu and other metal dopants (Ag , Y , Mn , Al ) were prepared by green synthesis using glucose as a capping agent. The properties of the nanoparticles were investigated using x-ray diffraction, scanning electron microscopy, Fourier transform infrared spectroscopy, ultraviolet-visible spectroscopy and photoluminescence spectroscopy. The XRD pattern exhibited a zinc-blend crystal structure at room temperature. The particle sizes of the nanoparticles were determined to be between 9.30 nm - 31.5 nm. The UV-Vis absorption spectrum showed that the optimum excitation wavelength was at 330 nm and the optical bandgaps were determined to be significantly different, ranging from 5 eV to 5.5 eV. The photoluminescence spectrum of ZnS:Cu nanoparticles show an orange visible spectrum.
+
+
2+
3+
Future Work
The parameters for PL and XRD can be standardised and the same machine could be used to minimise systematic error caused by instrument for future investigation. The difference in bandgap between the 2 ZnS:Cu could be investigated in relation to its particle size, using SEM which should be done for all samples to obtain a more accurate particle size. As a final application, an LED screen could be constructed using the nanoparticles as the luminescent layer.

An example of QD-LED structure. (Amini et al., 2015)
HTL: Hole transport layer
ETL: Electron transport layer
ITO: indium tin oxide
References
Amini P., Dolatyari M., Rostami G. & Rostami A. (2015). High Throughput Quantum Dot Based LEDs, Energy Efficiency Improvements in Smart Grid Components, Prof. Moustafa Eissa (Ed.), InTech, DOI: 10.5772/59092. Retrieved March 13, 2017 from http://www.intechopen.com/books/energy-efficiency-improvements-in-smart-grid-components/high-throughput-quantum-dot-based-leds
​
Bagher, A. M. (2016). Quantum Dots Applications. Sensors and Transducers,198(3), 37-43. Retrieved October 29, 2016, from https://www.researchgate.net/publication/299505883_Quantum_Dots_Applications.
​
Cadmium Selenium; MSDS No. 1306-24-7; n.d. Available from Plasmaterials Inc. http://www.plasmaterials.com/msds/CdSe.pdf (accessed 29/10/2016)
​
Coe-Sullivan, S., Steckel, J.S., Woo, W.K., Bawendi, M.G. & Bulovic, V. (2005). The Application of Quantum Dots in Display Technology. Material Matters, 2(1), 1117. Retrieved October 29, 2016, from http://www.sigmaaldrich.com/technical-documents/articles/material-matters/the-application-of.htm
​
Iranmanesh, P., Saeednia, S., & Nourzpoor, M. (2015). Characterization of ZnS nanoparticles synthesised by co-precipitation method. Chinese Physics B, 24(4)
​
Peng, W., Cong, G., Qu, S. & Wang, Z. (2005). Synthesis and photoluminescence of ZnS:Cu nanoparticles. Optical Materials, 29, 313-317. doi:10.1016/j/optmat.2005.10.003
​
Punjabi, K., Choudhary, P., Samant, L., Mukherjee, S., Vaidya, S., & Chowdhary, A. (2015). Biosynthesis of Nanoparticles : A Review. International Journal of Pharmaceutical Sciences Review and Research, 30(1), 219-226.
​
Senapati, U. S., Jha, D. K. & Sarkar, D. (2013). Green synthesis and characterization of ZnS nanoparticles. Research Journal of Physical Sciences,1(7), 1-6. Retrieved October 27, 2016.
​
Walling, M. A., Novak, J. A. & Shepard, J. R. E. (2009). Quantum Dots for Live Cell and In Vivo Imaging. International Journal of Molecular Sciences, 10(2), 441–491. Retrieved March 13, 2017 from http://doi.org/10.3390/ijms10020441
​
ZióÅ‚czyk, P., Miller, E. & Przybyt, M. (2014). ZnS Cu-doped quantum dots. Biotechnology and Food Sciences, 78(1), 53-69. Retrieved October 27, 2016, from http://repozytorium.p.lodz.pl/bitstream/handle/11652/620/ZnS_Cu_doper_quantum_Ziolczyk_2014.pdf?sequence=1
​
​
​
​
​
​
​
​
​
​
​
​
​
​
The authors gratefully acknowledge Hwa Chong Institution and Dankuk University for providing laboratory facilities and necessary resources, without which the research could not have been completed. The authors also acknowledge National University of Singapore (NUS) for the SEM images and XRD measurements.
​
The authors would like to thank our project mentors Kong Chiak Wu, Mr. from Hwa Chong Institution who provided insight and expertise that assisted the research.
​
The authors would also like to thank Lim Cheng Fui, Mdm. from Hwa Chong Institution (Science Research Centre) for coordinating with NUS and for her assistance during experimental research.
Materials and Methods
-
Sodium sulphide nonahydrate
(Na2S·9H2O)
​​
Respective metal nitrates:
-
Zn(NO3)2·6H2O
-
Cu(NO3)2·6H2O
-
AgNO3
-
Y(NO3)3·6H2O
-
Mn(NO3)2
-
Al(NO3)3·9H2O
​​
-
D-glucose (C6H12O6)
Reagents
Apparatus
-
Mortar & Pestle
-
Magnetic Stirrer
-
Centrifuge
-
Measuring Cylinder, Beaker & Flask
-
Water Bath
-
Incubator
Equipment
-
X-ray diffraction (XRD) – determine crystal structure and estimate size of nanoparticles
​
-
Scanning Electron Microscope (SEM) – estimate size of nanoparticles
​​
-
Fourier Transmission Infrared Spectroscopy (FTIR) – determine if glucose is successfully added as functional group
Synthesis
Zn(NO3)2·6H2O was dissolved in 100 ml of deionised water to obtain 1.00 mol dm Zn(NO3)2 (aq). 1.00 g of Cu(NO3)2 and 1.00 g Mn(NO3)2 were dissolved in the same solution. Na2S·9H2O was dissolved in 100 ml deionised water separately to obtain 1.00 mol dm Na2S (aq). 1.00 mol dm Na2S aqueous solution was added dropwise with continuous stirring to 1.00 mol dm Zn(NO3)2 aqueous solution doped with Cu and Mn . A white colour solution was formed after 15 h of continuous stirring. 1.00 mol dm glucose solution was added dropwise to this white colour solution. The resultant solution was placed in a water bath at 70°C for more than 6 hours. Precipitates obtained were centrifuged with 3500 rpm for 15 minutes. The final products were dried at 50°C for more than 4 hours in an oven and then crushed to fine powder.
​​
The procedure was repeated, replacing Mn(NO3)2 with AgNO3, Y(NO3)3·6H2O and Al(NO3)3·9H2O respectively. The above synthesis process can be summarised by the chemical equation:
​
Zn(NO3)2 + Na2S → ZnS + 2NaNO3
​
Capping Agent: Glucose
2+
2+
-3
-3
-3
-3
-3
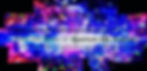
Quantum Dots
ZnS nanoparticles are quantum dots, a semiconductor crystal of nanometre dimensions (2-10 nm) with distinctive conductive properties determined by its size. The narrow bandgap of quantum dots which are semiconductors absorb electromagnetic radiation from UV to visible range, exciting the electrons. When the electrons of the quantum dots return to ground state, energy is released as light energy.
Quantum dots exhibit quantum confinement effect, because of their radius which is smaller than the characteristic Bohr exciton radius, so the carriers in all three directions confined and the density of states will change with the size of nanoparticles. The density of states (DOS) refers to the number of different states at a particular energy level that electrons are allowed to occupy.
Effect of doping different metals on the photoluminescence of ZnS nanoparticles
Results and Discussion
Bohr excitation radius: Distance between electron-hole pair
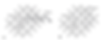

Relationship between size of nanoparticle, optical bandgap and colour of light emitted.
Literature Review
There has been a growing interest in the use of quantum dots (QD) in recent years with the development of nanoparticles for their applications in QD displays and medical applications such as bioimaging.
​
Quantum dot light emitting diodes (QD-LEDs) have 40% purer colours, lower power consumption, lower cost of manufacturing than OLED and are ultrathin, transparent and flexible to realise new display and lighting forms not possible with existing technologies (Bagher, 2016). Theoretically, QD-LEDs also have a combination of luminous efficiency, stability and lifespan exceeding other display technologies. (Coe-Sullivan, Steckel, Woo, Bawendi & Bulovic. 2005).
​
ZnS quantum dots also have extensive applications in bioimaging. As a biological marker, it is biocompatible being non-toxic, has improved photostability, simple and routine fabrication protocols and uniform spectral profiles which are suitable for in vivo imaging and tracking (Walling, Novak & Shepard, 2009)
​
The most commonly used QD include these composed of CdSe, ZnSe and ZnS. The luminescence of QDs is caused by the quantum confinement effect and defects in the crystalline structure of QDs. Since the bandgap in semiconductors is relatively narrow, they absorb light in the ultraviolet and visible spectrum. The smaller the quantum dot, the wider the energy bandgap, hence affecting the wavelength of the emitted light. Doping metal ions to quantum dots to form additional energy levels can change the photoluminescence and ultraviolet emission spectrum of the QDs (ZióÅ‚czyk, Miller & Przybyt, 2014).
​
Among II-VI class inorganic semiconductor nanomaterials, CdSe has emerged as the most commonly used QD due to its ability to emit light from almost the whole visible spectrum from 470nm to 640nm just by changing its size (Coe-Sullivan et al., 2005). However, Cd and Se compounds are carcinogenic and poisonous respectively which can leave serious health hazards if it leaks into the environment. (Cadmium Selenium; MSDS No. 1306-24-7). ZnS is also known to be more chemically stable and technologically better than other chalcogenides like ZnSe (Peng, Cong, Qu & Wang, 2005).
​
Despite rapid advancement in techniques for fabrication of nanoparticles in the last 2 decades and vast potential of quantum dots for various applications, research on the characteristics and photoluminescence multiple doped ZnS nanoparticles is still limited. The use of chemical based methods to produce ZnS nanoparticles on large scale have also raised concerns over potential environmental harms, thus further research has to be made to look into more eco-friendly methods to synthesise ZnS nanoparticles (Senapati, Jha & Sarkar, 2013).
Objective and Hypothesis
2+
2+
+
+
3+
This research aims to
-
synthesise ZnS nanoparticles co-doped with Cu , and Mn , Ag , Y or Al respectively;
-
investigate their respective UV-Vis spectrum to determine optical bandgap and optimal excitation wavelength;
-
and thus investigate their respective photoluminescence (PL) spectrum.
It was hypothesised that
-
the PL spectrum of doped ZnS nanoparticles will have additional peaks for corresponding dopant and;
-
additional dopants will result in significant difference in optical bandgap of nanoparticles.​
​
Independent Variable: Metal dopants - Mn , Ag , Y or Al
Dependent Variable: Size, PL spectrum, UV-Vis spectrum of ZnS nanoparticles
​
Constants:
-
1.00% (wt) Cu -doped ZnS nanoparticles;
-
pH, temperature and pressure of synthesis;
-
Concentration of metal dopants
2+
+
+
3+
2+
Conclusion
ZnS nanoparticles have been successfully synthesised via green route. The chemical bonding, structural properties and particle sizes of the samples were characterised by FTIR, XRD and SEM. The XRD patterns indicated that ZnS nanoparticles with cubic zinc blende structure have been synthesised despite impurities. The SEM images and XRD calculations revealed that the ZnS nanoparticles have an average size between 9.30 nm – 31.5 nm. The UV spectrums showed that the optimal excitation peak were at 330 nm and the optical bandgaps were calculated to be between 5 eV - 5.5 eV, which were found to exhibit significant difference with different metal dopants. An overall red shift was observed for the PL spectrums obtained by Bugil. The initial hypothesis that the PL spectrum of doped ZnS nanoparticles will have additional peaks for corresponding dopant were, however, inconclusive.